In this blog, we’ll answer:
- What types of battery aging exist and what are the main influencing factors?
- What are the characteristics of energy trading and frequency regulation use cases?
- How do use cases impact battery aging?
- What does this mean for the energy management system?
Warranty conditions are often given in terms of years and cycle numbers. Analogously, battery aging can be divided into calendar and cycle aging. Let's go step by step and apply two simple rules of thumb:
- Everything that is “high” harms the battery.
- The dependencies are non-linear, with high values being much worse than low values.
How to Optimize Battery Lifetime During Calendar Aging
Calendar aging occurs over time even when the battery is not in operation. It’s like how we age while we sleep. The two important factors to consider are the state of charge (SOC) of the battery and the temperature of the battery while at rest.
Accounting for State of Charge (SOC)
High states of charge (SOCs) will reduce lithium-ion battery life, and small increases in SOC can significantly speed up capacity loss, especially in the upper range of the SOC. This is primarily due to the formation of certain passivation layers on the anode.
Figure 1 depicts the relationship between high SOC and battery aging. In this experiment, several battery cells of the same type were stored at different SOCs and an elevated temperature of X °C. The values above 80% SOC show significantly greater reductions in capacity, while long lifetimes are achieved at the lower and medium states of charge. Although the quantitative correlation depends on the cell chemistry and the specific cell, this tendency applies to lithium-ion technologies in general.
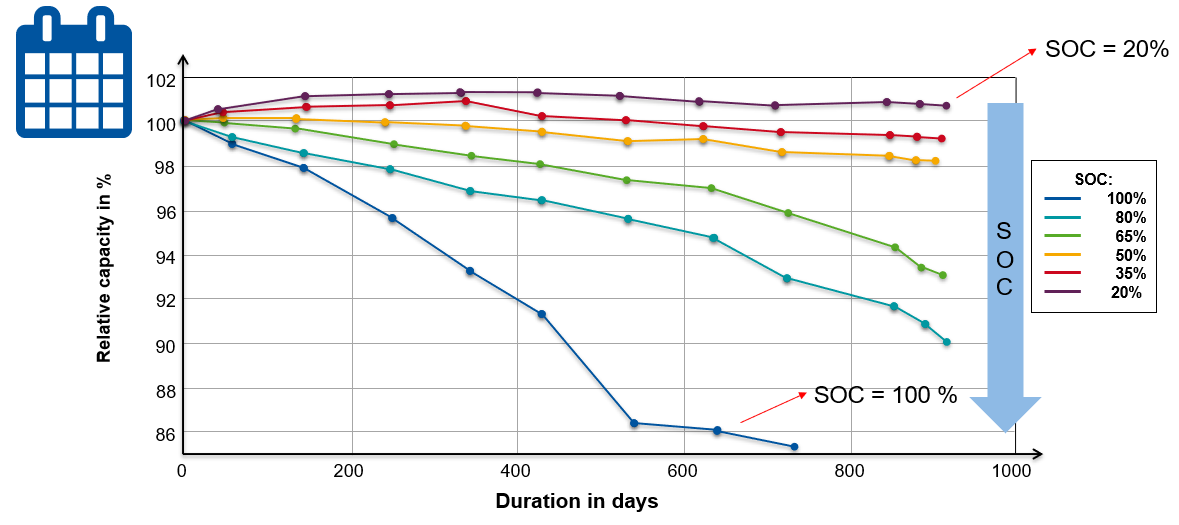
Lower Temperatures Are Ideal for Battery Lifetime
High temperatures speed up chemical degradation within battery cells, leading to faster capacity loss. Like high SOCs, the interconnection between temperature and aging is non-linear with small increases leading to significant reductions in lifetime. As a rule of thumb, one can say that an increase in temperature by 10 Kelvin can halve a battery’s lifetime. Conversely, lower temperatures slow down the aging processes.
Improving Battery Life Through Optimal Cycle Aging
Cyclical aging is wear and tear that results from active battery use, much like how we age faster when we are under stress working long hours.
Limit Depth of Discharge (DOD) by Charging Frequently
The depth of discharge is a percentage of that battery’s total capacity that is used for a specific operation cycle. While full charges and discharges would correspond to a DOD of 100%, charging a battery from 50% to 70% and discharging it back to 50% would correspond to a cycle DOD of 20%, exactly as going from 50% to 30% and back to 50%. High DODs, for example, cause higher mechanical stress when lithium ions intercalate in electrodes and electrochemical reactions, accelerating aging.
Like SOC and temperature, the impact of DOD on battery lifetimes is not linear, as shown in Figure 2. This experiment shows battery cells of the same type that were continuously charged and discharged under the same environmental conditions. The average state of charge was kept identical to ensure similar calendar aging. So-called equivalent full cycles are shown on the X-axis. These are calculated from partial cycles and can be seen as capacity throughput to bring results to the same scale. For example, two partial cycles of 50% correspond to one EFC. Analogously, five 20% cycles or ten partial cycles of 10% would be depicted as one EFC. While the smaller DODs achieve long lifetimes, the higher DODs lead to high-capacity losses.
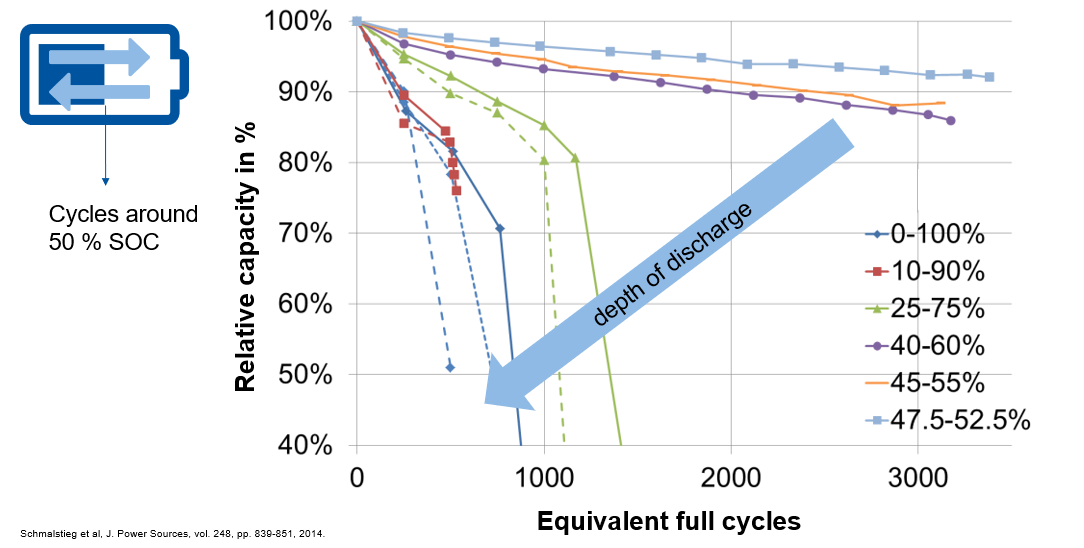
Slow Down Current Rate (C-rate)
The current rate is a measure of how quickly the battery charges or discharges. It is calculated by dividing the current by the nominal battery capacity. Let’s do some examples:
If a 300 Ah cell is discharged with 300 A, the C-rate is 1 C ( = 300 A / 300 Ah), if the discharge current is only 150 A, the C-rate is 0.5 C (= 150 A / 300 Ah) in turn.
First, the C-rate capability is highly dependent on the specific cell. Second, the C-rates that occur depend on the system design. For stationary large-scale storage, typical C-rates are below 1 C, as most of the systems are designed as one—to four-hour systems, meaning the duration in which they can be charged or discharged at full power.
High C-rates accelerate battery aging, again in a non-linear relationship. This is one area where we will break our rule that “everything that is high harms the battery, because it is especially low temperatures in combination with high charging currents that accelerate aging in the form of lithium-ion plating. This is a reason for capacity loss and possibly unsafe operational states.
To simplify what we’ve covered above: Batteries age like rubber bands. Keeping a rubber band stretched at maximum (high SOC) for a long time (calendar aging) will make it brittle and prone to tearing. Likewise, repeatedly (cycle aging) stretching it too quickly and widely (high DOD and C-rate) will also cause it to tear much sooner than if it is only stretched gently.
Now, let's explore how different use cases affect both calendar and cycle aging in grid-connected large-scale storage systems.
Use Cases: Spot Market Trading and Frequency Regulation
As mentioned in the beginning, different use cases affect battery lifetimes in different ways, so understanding and monitoring these effects is imperative.
Spot Market Trading
Spot market trading involves buying and selling electricity in real-time to capitalize on price differences. Nowadays, typical storage system designs for multi-use applications are in the 1 to 4-hour range. Let’s assume a 2-hour system for the examples in Figure 3 below. This means the maximum possible C-rate is 0.5 C for this system layout.
Intraday Trading
Because revenues go hand in hand with energy throughput on the spot market, many cycles with rather high DOD can occur, as shown in Figure 3.

Frequency Regulation
Frequency regulation is a critical function in maintaining grid stability. It helps balance the grid frequency by managing the difference between electricity supply and demand. Because the frequency is quite stable in most mature energy markets, the power to be provided is very small. This use case usually involves operating the battery within small depth of discharges (DODs) and around mid-state of charge (SOC) ranges, which proves to be beneficial in minimizing battery life. Note that sometimes the market product is bidirectional (frequency containment reserve), and sometimes it is unidirectional (automatic frequency restoration reserve).
Comparative Analysis of Use Cases' Effect on Aging Parameters
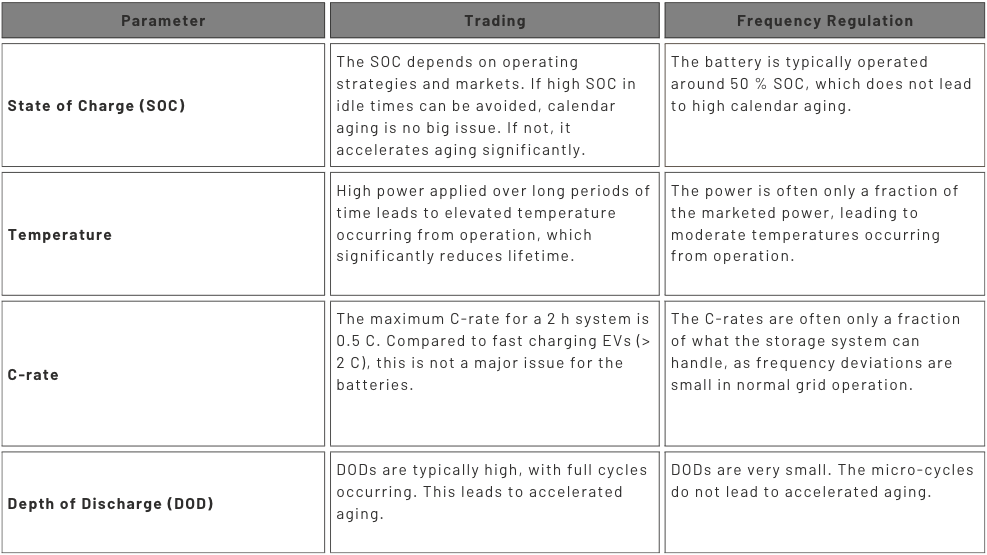
Key Takeaways for Energy Management
By understanding how different use cases impact lithium-ion battery lifetime, appropriate operational strategies can be implemented, enabling stakeholders to maximize the lifespan and performance of grid-connected large-scale battery storage systems.
Aim for Low SOCs at Rest:
For frequency regulation, this is usually done by default. In terms of energy trading, idle times at high SOCs should be kept as short as possible.
Avoid High Temperatures:
For frequency regulation, this is usually done by default. In terms of providing high power over a long period in energy trading, a good cooling system is needed, and resting periods should be included in trading strategies.
More Frequent, Smaller Charge Cycle for Lower DODs:
Again, frequency regulation avoids high DODs by default. In terms of trading, opt for multiple smaller cycles over one large cycle; two 50% cycles are better than one 100% cycle.
Understand C-rate Influence:
For many cells, a C-rate of 0.5 C (2 h system) or 0.25 C (4 h system) is no major problem. For others, smaller C-rates can be a challenge. So, be sure to understand the C-rate's influence and consider it while implementing your energy management system.
Battery aging is complex, and every cell ages differently. More still, the spread between the worst and the best cell is increasing rapidly over time.